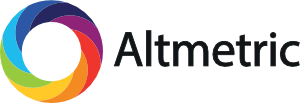
How did the earliest and largest clusters of galaxies form? In episode 88, Arianna Long from the University California – Irvine talks with us about her research into the emergence of massive dusty star-forming galaxies that developed billions of years ago. Her article “Emergence of an Ultra-Red Ultra-Massive Galaxy Cluster Core at z = 4” was published on July 31, 2020 with multiple co-authors in The Astrophysical Journal and first submitted to arXiv as a preprint on March 30th 2020.
Websites and other resources
-
- Arianna on Twitter, including her thread on this study
- Arianna’s article “The Curious Case of Dusty Star-Forming Galaxies” for the Astronomical Society of the Pacific
- Arianna’s Women in Natural Sciences (WiNS) Award from UCI
- African American Women in Physics’ website and on Facebook
Bonus Clips
🔊 Access bonus content here.
Support us for as little as $1 per month at Patreon. Cancel anytime.
We’re not a registered tax-exempt organization, so unfortunately gifts aren’t tax deductible.
Hosts / Producers
Doug Leigh & Ryan Watkins
How to Cite
Music
What’s The Angle? by Shane Ivers
Transcript
Arianna Long: What we really want to do as astronomers is we really want to push back the age at which we can study these protoclusters – so baby galaxy clusters – and really try to capture that moment in time when they’re forming most of their stars.
Ryan Watkins: This is Parsing Science, the unpublished stories behind the world’s most compelling science, as told by the researchers themselves. I’m Ryan Watkins.
Doug Leigh: And I’m Doug Leigh. Today in Episode 88 of Parsing Science, we’ll talk with Arianna Long from the University of California Irvine, about her research into the emergence of massive dusty star-forming galaxies, which developed billions of years ago. Here’s Arianna Long.
Long: Hi, I’m Arianna Long. I am a doctoral candidate at the University of California Irvine, studying physics and astronomy. I grew up in the Florida Keys for my elementary years, where I definitely fell in love with the night sky, but also the ocean abyss. Just the … I guess the entirety of the depths of the universe really fascinated me. And then I moved to Maryland, in middle school, roughly. And then I was really good at sciences. Love the science, love math. So I ended up going to school to actually be a math teacher at Towson University, which is a really great teaching school in the state of Maryland. While I was there, I realized I actually really, really liked the science part. But I was pretty progressed in my degree by the time I figured that out. I am a first-gen student. And so you know, no one in my family actually went through the Ph.D. process or did any science in college. So I didn’t even know really what that meant. So I did a little bit of research and mathematical modeling in my senior year. But again, it was a little late to kind of apply to grad school. And to be honest, I wanted to work a little bit and make some money. So I moved out to California and worked, actually, as a business consultant for about a year and a half. And it was interesting, but I couldn’t really fight the fact that in my heart, I felt this tug towards science and exploration. So I ended up applying to a master’s program since I didn’t have an explicit physics background. I knew I wanted to go back to school for astrophysics. It was one of those things: being in LA, everybody’s chasing their dreams, the musicians, the actors, and was like, “Cool, I would like to chase my dreams.” And so, I wanted to go back to school to study astrophysics. But my physics background wasn’t a strong. Math is not physics. So I went and got a Master’s – a research based Master’s – at Cal State Los Angeles: shout out. And then I bridged right in after graduating, I went straight into my doctorate degree. And now I study massive galaxy evolution, all the things that create them and tame their growth in the universe.
Watkins: Though we might not realize it from our local perspective in space and time, most galaxies aren’t alone in the vast expanse of space, but are connected to one or more other galaxies by gravity, or the bending of space-time. Regardless of what holds them together, these groups of galaxies can be small – such as two galaxies orbiting each other – or large, with thousands of galaxies extending for more than 10 million light years. We began our conversation by asking Arianna to tell us more about the general characteristics of galaxy clusters, as well as those that she studies in particular.
Galaxy clusters and protoclusters
Long: Galaxy clusters – most of what we know about them, and our discovery of them – are when they’re fully formed. So there are these groups of hundreds to thousands of galaxies dancing around each other, gravitationally bound. And when we see them, usually, it’s in the local universe. So when the universe is, you know, 11, 12, 13, nearly 14, – which is where we are – billion years old. And so they’re fully formed. They’re usually full of these really, really massive, but dead galaxies. And that’s fascinating to us. And what I mean by dead is … galaxies, when they’re alive and active – kind of like the Milky Way – it means it’s still forming stars. And it still has gas that it’s turning into these stars. It has this beautiful bluish color from UV light, which is coming from younger hot stars. But when it’s dead, it’s no longer forming then, and it turns like this orangish-yellow. And actually, we refer to them in the field as “red and dead” galaxies. And so you might hear me say that a few times. And so these galaxy clusters are actually predominantly full of these “red and dead,” actually elliptical galaxies instead of spiral. They’re these big, spheroidal dead galaxies. And that’s really, really fascinating to us. Because when we study these galaxy clusters out to a certain age in the universe, we find that in general, this dead population make up is pretty pervasive throughout clusters, all the way up to the time when the universe was about only half of its current age. Which leads us to believe that cluster galaxies they must have formed most of their stars earlier in the universe, reaching masses equivalent to – or even exceeding that – of the Milky Way, but up to 10 billion years sooner. And so, what we really want to do as astronomers is we really want to push back the age at which we can study these protoclusters – so baby galaxy clusters – and really try to capture that moment in time when they’re forming most of their stars. When the clusters are actually full of star-forming blue, beautiful galaxies. We want to know what’s going on. We want to know how they get as big as they get. And we want to know the actual mechanisms to which they fall in together into this huge, huge dark matter halo.
[ Back to topics ]
The life cycle of galaxy clusters
Leigh: The evolution of galaxy clusters plays out over billions of years, with inner galaxies being birthed, merging or dying away over hundreds of millions of years. Since these clusters evolve very slowly, they can retain an imprint of how they were formed. And if we can interpret the data correctly, they can give us a better understanding of the history and structure of their formation. Since Arianna’s research examines the evolution of these galaxy clusters, Ryan and I asked her to describe their life cycles.
Long: General galaxy evolution – massive or not – usually starts with the collapse of a highly dense cloud of gas. All the pressure at the center of that highly dense cloud turned into stars, more stars pop off, and then it turns into a galaxy. Then you might have some mergers of smaller or larger galaxies. And it keeps building, keeps building, until there’s no more influx of gas. Gases is like the life source. And so once there’s no more gas readily available to turn into stars, that is when the galaxy starts to quote-unquote, die. And it’ll run out of its fuel. And eventually, all the young stars will become old, which is how they turn yellow and orange and red. And then everything eventually turns to black holes. And then you get black hole decay. And that’s a whole string theory thing. What is really difficult about massive galaxy evolution, and starburst, and things like that is there’s a lot of degeneracies. So a massive galaxy: it probably started off pretty big on its own. So it was probably a pretty early on collapse of a highly dense gas cloud. But there was also probably a lot of mergers. So lots of mergers of major and minor sizes. And what I mean by that is, “major” is usually a galaxy that’s of comparable size. So any ratio of like one to three or more, I guess. And then a “minor” merger would be like a satellite galaxy, like a small one kind of merging into the main galaxy.
So massive galaxies, what’s really interesting about them is the models – at least as far as we know – the models support this idea that there was likely a major merger at some point, which actually kind of triggered the sudden uptick in star formation. All of this fresh gas from two galaxies colliding. Instead of kind of slowly funneling in from spiral arms, like we believe it does, with like Milky Way and spiral galaxies, kind of taking its time funneling gas here and there. The actual sudden collision of a ton of gas from two galaxies causes a sudden uptick in stars forming. And so it actually looks probably more like a, what they call, like a “blue nugget.” So instead of again, a really pretty spiral, you probably have this blue spheroidal thing because two galaxies merge and everything’s kind of pushed around. It’s not as settled into a plane. And so it looks like this blue nugget’s really dusty, likely. And there’s probably what’s called an AGN – an active galactic nuclei – which is a supermassive black hole that is actively accreting dust and gas nearby. And there’s another component, these black holes actually sometimes have columnated -so twin jets coming out of them – that inject a lot of energy into the atmosphere and might even heat up and blow away some of the cool gas necessary to to continue forming stars. So there’s this cycle of feeding and feedback, that actually will maintain the growth for a little bit, but for a short period of time for these massive galaxies. So, “short” being on the order of several hundred million years instead of a few billion years, or 10 billion years. And then eventually, it’ll run out of that gas unless another merger comes in, like that could jump it back up into star-forming mode again. But eventually those mergers will slow down, and it’s Halo – so its surrounding dark matter body – will actually be full of like hot gas and hot material that will even prevent more gas from accreting on. And it reaches this kind of maximum mass threshold that a lot of people study, where essentially, there’s this threshold of mass that a galaxy could meet. And then once it meets that mass, it has accreted so much dark matter and baryonic matter that the pressure and all the other feedback from the stars and the AGN causes the halo to be hot and shock heated. And so any gas that may try to come in is actually prevented or heated or stripped away. So it essentially kind of like starves itself. And then it turns into that big, big red and dead spheroidal that we see: those elliptical galaxies.
[ Back to topics ]
Redshift
Watkins: From the longer waves associated with red to the shorter waves associated with violet, we humans see the universe through a fairly narrow spectrum of visible light waves. And in 1842, the work of Christian Doppler expanded our knowledge of electromagnetic radiation, with longer or shorter wavelengths that are outside the boundaries of our visible spectrum. On Earth, as visible objects move away from an observer, their wave links lengthen. While our eyes don’t see any visible change in color, this lengthening of the wave is referred to as a “redshift.” And astrophysicist use this principle to study the evolution of galaxy clusters, as Arianna explains next.
Long: We use redshift to actually denote the place in time at which we study these galaxies. It has to do with the fact that the light from these galaxies was emitted so long ago that the universe was still accelerating and stretching out in space-time. And so their light has been shifted, has been stretched out, to lower energy regimes, which we refer to as “redder.” And so we push out to really high redshifts to observe early galaxies, some of the first galaxies, some of the first stars, and some of the first protoclusters. And when we want to see – if we want to capture protoclusters, or galaxy clusters in formation – that means we have to push even further than we would expect. Because they’re usually, again, already, quote-unquote, red and dead pretty early on. And so what we did is, we found a couple of different protoclusters, but they all looked different. And depending on the astronomer, and how you define a protocluster, it’s highly debatable. A big part of that has to do with what part of the energy spectrum that you spend your time studying. I study the infrared portion of the spectrum. I do study all parts of the energy spectrum – or the electromagnetic spectrum – like most people do in my field, but my main interest is the infrared part. And so, when we’re actually pushing all the way back, and we’re finding these protoclusters, there’s kind of two different kinds that we’re finding. One is a protoclusters full of normal star-forming galaxies. So normal star-forming galaxies, are actually the most common type. Our Milky Way is one of them. And it’s a galaxy that forms about 1 to 3 to 10 solar masses per year. It’s pretty average, it’s like a steady state, kind of like a metaphorical “tortoise.” So over the course of 10 billion years or so they form their stars slowly and steadily remaining blue and disk-like, depleting their fresh gas reservoir at a leisurely pace. But then there’s this other type of galaxy, we refer to them, often as “starburst” galaxies, and I like to think of them as the metaphorical “hares” in galaxy evolution. So in maybe a few hundred million years at most, these galaxies kind of burst into existence. They form as many stars as rapidly as possible, and then they run out of fuel. They live fast, die young. And a lot of astronomers – based on models – comparisons of their actual size, think that they’re the best candidate ancestors for the big, massive dead … red and dead galaxies that we see in clusters today. The problem is, [in] starburst galaxies, the actual rate of star formation also comes with a lot of stellar death. And in supernova, there’s a lot of dust production, and dust actually obscures a lot of light, mostly in the UV and optical spectrum. And historically, astronomy has been performed in the optical regime, because that is the energy wavelength that we are sensitive to with our eyes. But the starburst galaxies, the light that is emitted by all these young hot stars – this ultraviolet light – is actually absorbed by the dust in between. And so while we can’t see in the optical that dust actually re-radiates in the infrared. And so you can actually look at a picture – there’s some really cool pictures online, if you look up “infrared universe” versus you know, “optical universe,” there’s some really great tools online to actually see the difference in the night sky or – in any given galaxy, different energy regimes – and you’ll see that galaxies with a lot of star formation and – these like stellar nebula these stellar nurseries – they are difficult or almost impossible to see in the optical, but they light up beautifully and you can actually peer through them in the infrared.
[ Back to topics ]
The Distant Red Core (DRC)
Leigh: The precursors of galaxy clusters, known as protoclusters allow researchers like Arianna to explore some of the earliest times in the evolution of our universe. To the best of our knowledge, however, these are rare events. And given the vastness of space, how far back they occurred in the past, and the volatility of these processes, which often create huge clouds of dust that can obscure evidence of their development, Arianna required some of the best tools in astrophysics to conduct her research … and more than a little bit of patience. Up next, she tells me about how she and her colleagues went about studying a particular protocluster rich with dusty star-forming galaxies known as the Distant Red Core.
Long: When you’re looking for protoclusters, what you’re looking for is essentially like an over-density in the field. You’re looking for a location in the sky, and in redshift space. So we have to consider age and time. So you’re looking for a location in volume – or in space and time – that has, I guess, like an increase, or surprising increase, a significant increase in a population of galaxies. And then you have to do a bunch of follow up studies to actually prove that they’re all at the same space and time, and prove that it’s actually significantly over a dense compared to say field galaxies, which are kind of just hanging out by themselves. And so a lot of astronomers would use optical telescopes or UV telescopes to find over-densities of these normal star-forming galaxies. So very blue, not as dusty, pretty steady state. But when they do that they’re, again, they’re biased against seeing these more starburst galaxies, which we’re finding are more and more common earlier in the universe. And again, if you remember, I said that they’re really great candidates for forming really, really big elliptical galaxies really fast, really early. Some astronomers have looked at infrared maps using mostly the Herschel Space Telescope or Herschel Space Observatory, to look for these really, really bright infrared sources. But the problem is -there’s always problems, right – there’s biases and issues with telescopes. So there’s a lot of trade offs, sometimes you can have … you can look at a bigger part of the sky, but maybe you lose some resolution. The Herschel space observatory was pretty low resolution. And so you could find all these beautiful infrared bright galaxies that you couldn’t see with optical telescopes. But they were so blurry – and possibly even blended with many nearby galaxies – that we couldn’t really draw as many conclusions as we wanted to. And actually, there’s been a flurry of follow up papers in the past five years, with the ALMA Observatory in Chile, that has a better resolution [and] that has actually broken apart a lot of Herschel sources into multiples. And so that actually brings me to where we are, which is if we want to look for over-densities of starbursts in the universe, then we need to use infrared telescopes to do so. And we need to actually perform a lot of follow up observations with telescopes to have better resolutions to actually separate different components.
And so the Distant Red Core is a source that was actually identified as a single source, and the Herschel Space Observatory image was a big blob. And it was expected to be around redshift four. So about when the universe was 1.5-ish billion years old: it’s pretty far back. And some of my colleagues have performed some follow-up observations with these fancier, more sharper telescopes like in Chile, and actually found that this one source resolved into about 11 individual starburst galaxies. Which is fascinating. And so, this discovery paper came out in 2018, detailing out “hey, we have this protocluster core,” is what they’re calling it. And the 11 galaxies are in a volume that’s even more dense than our own local group. So it’s these 11 galaxies in this really enclosed volume, forming something wild like 14,000 solar masses per year as a total. So they’re rapidly, rapidly forming, there’s actually some really incredible merging activity that we see. So, when you’re in a close environment like that, it’s expected that galaxies are going to merge and create crazy messes, you know, pulling out stars. But what they saw in 2018, was just some blobs. And they were able to confirm that they were at this age, and that they were individual galaxies. But what they couldn’t do is they couldn’t weigh the protocluster or these galaxies. And so what I mean by way is most of the weight in a galaxy comes from dark matter: we know that part. But that part is hard to see and hard for us to infer for many reasons. And so the other way that we weigh them, is we use lots of simulations tied in with observational data, that ties relationships between the amount of stars that a galaxy has, and the amount of dark matter that it likely has. And then we get what’s called the halo mass, the total halo mass. And so these relationships, I will preface by saying that they are largely based on optical and UV observations. So these relationships are based on normal star-forming galaxies. Again, they are the most common so I understand why theorists end up working on those galaxies. But as someone who works on rarer objects, I’m a bit frustrated by that. And so the reason that’s frustrating is because starburst galaxies, because they’re forming stars so quickly, and they are anomaly in and of themselves, they actually tend to be more massive than normal star-forming galaxies no matter their age. So if you compare a starburst galaxy and a normal galaxy – when the universe was half its age, so like seven billion years old – statistically speaking, it’s more likely that the starburst galaxy weighs more is more massive, has more stars. And so these relationships between dark matter and stellar mass are skewed. So if you go ahead and take that stellar mass of a normal – of a starburst galaxy – it’s actually possible and this is what happened, that your galaxy is so massive, it doesn’t even lie on the relationship. That relationship doesn’t even extend to how massive your galaxy is.
[ Back to topics ]
Requisitioning the Hubble Space Telescope
Watkins: Launched in 1990, the Hubble Observatory was the first major optical telescope to be placed in space above the distortion of the atmosphere, far above rain clouds and light pollution. Hubble has an unobstructed view of the universe, which was important for getting images of the Distant Red Core protocluster, Doug and I were curious to hear how one goes about requisitioning the Hubble Space Telescope to take pictures of a particular part of the universe for your research.
Long: I wrote a proposal for the Hubble Space Telescope a couple years ago to study some redshift four starburst galaxies: just a general sample. And serendipitously, while I was waiting for the proposal to come back, a paper was published on this protocluster after that was discovered: the Distant Red Core [at] redshift four. And then I got the proposal I was awarded, I was very excited. And I got a picture of this protocluster using the Hubble Space Telescope. And that’s important because the Hubble Space Telescope sees primarily in the UV and optical regimes – a little bit of near infrared – but mostly the UV and optical. And again, protoclusters at high redshifts have really only been observed in one or other regimes. So either just the UV optical or just the infrared: there’s only one. It’s really not as common, at least at these ages. So at lower ages – more local time – it is common to have multi wavelength perspectives. But at these high redshift, it’s so rare, and it’s still a budding field in that we really only have one type of picture for each protocluster. And that makes it really hard to compare different properties of the galaxies, because different energy regimes correspond to different properties. So this was the first time that we had a picture of the optical regime of an infrared bright starburst protocluster, which meant that we could see the stellar component instead of just the star-forming component. So in the infrared, we’re looking at the dust, the cold gas, the stellar nebula. We’re learning a lot about its birthing rates, things like that. But with the UV and optical data, we learn a lot about its history, because the UV and optical is where most stellar light emits adult stars. And so we learn a lot more about the star formation history. And then we can also weigh the galaxy. But the problem was, is when I went to go try to weigh them, they were off that relationship that I mentioned earlier, that would actually give me a halo mass based on the stellar light. They were considered so big – and not all of them, I would say was about four of them – were considered so massive that they might not even … it might not even be possible for them to exist. And I thought that was really interesting.
So I went and looked into some papers that did some simulations on the biggest possible allowable halos that could exist in this universe, assuming our understanding of the current cosmology is correct. And when you do that – and you actually combine all these halo measurements, and these weights – we found as a whole, the cluster may actually violate that prediction. So we might actually be in violation of the largest allowable halo in the universe. There’s big error bars, and lots of follow up studies need to happen. And of course, there’s a lot of assumptions that go into the relationship, again, as I as I mentioned earlier, that relates stellar mass and halo mass. So with all of that we have pretty large error bars, but it does sit nicely in that region of like, have a three sigma … like a very strong possibility of violating our current understanding of cosmology. And then you can exacerbate that by assuming that it’s very possible that there are normal star-forming galaxies nearby that we aren’t picking up. Normal star-forming galaxies have a little bit of infrared emission, the Milky Way definitely does just not as significant as starbursts. And so you need deeper observations that stare at the sky for longer to pick them up, and we don’t have those. And so, in the picture that we see with the optical wavelengths, we actually see a lot of galaxies nearby are starburst galaxies, but we don’t have redshift information for them. And so there’s this issue of projection. Like if you look out into the universe, you’re seeing the past, but it’s all collapsed into 2D. We do definitely see lots of other galaxies nearby – and it looks like there are probably lots of star-forming galaxies nearby – but we need more observations to prove that. But I say that because if you include the fact that it’s likely that there’s normal star-forming galaxies nearby, then the mass is even bigger, and the halo mass is even bigger. So even though our error bars are large, it’s likely that they’re still an under-estimate. So these dusty starburst protocluster cores, a second one was also reported and published in the same year as mine, the SPT protocluster. And the fact that there’s now two and people are looking for even more – assuming that this is more commonplace – having these massive dusty protocluster cores is really kind of throwing a wrench in our understanding of massive galaxy formation and galaxy cluster formation in the early universe.
[ Back to topics ]
Galaxy clusters in the DRC
Leigh: Along with existing data from three other terrestrial telescopes, Arianna and our colleagues use data from both the Hubble and Spitzer Space Telescopes in order to study the Distant Red Core. They confirmed a population of massive galaxies in a place when the universe was only 1.5 billion years old. We’ll hear what Arianna had to say about the study’s findings, as well as what still unresolved questions remain, after this short break.
[ad: Altmetric]
Leigh: Here again is Arianna Long.
Long: We have spectroscopic information on all 11. So we know that they’re in the same physical location, roughly speaking, in the universe at the same time. And so I feel confident in that. So the first four, they were the brightest in the infrared, they’re the most star-forming. But all 11 were confirmed. So that’s fine, I can handle that. But when you actually look at their stellar component – their stellar light; so using the Hubble telescope – you see across the board, really, really confusing things. So there were two objects – DRC 5 and 11 – were clearly there was only one counterpart in the Hubble image Almost every other image, or every other galaxy, either didn’t have a Hubble counterpart, or they have multiple potential counterparts. For example, DRC 1: there’s like clearly like a big blob, and then a little blob that are separate. And to someone’s untrained eye – and if you were doing some more traditional counterpart matching techniques – you would only choose the big one, because it was closest to the center of my bright, unresolved image, and it was the brightest. But I knew from one follow up study – just on this one object, just on the first one, not any of the other 11 – that in fact, both of these blobs belong together. They’re at the same redshift, and they’re going to be merging. Just one happens to be bigger than the other. And so with that information, I thought, “Okay, if that’s the case, for DRC 1 – and again, we’re looking at an over-dense environment – that it’s likely that … and it’s possible that … all 11 of these objects with multiple potential galaxy counterparts, they could be globally a part of the same system.” So then DRC 2 – which, again, is very bright and very secure – when you actually look at it and Hubble it breaks up … almost depending on how you define components … it breaks up into potential to 20 different components. But strong components would be about three or four. And it’s messy, it doesn’t look like a traditional galaxy blob that I’m used to seeing. And so, I would say that one, and DRC 7 and 8, were kind of the more difficult ones, because there’s clearly multiple galaxies. But it is possible, it’s … sure, statistically speaking, there is that 0.[0]1 or 1% chance that some of those objects aren’t at that same location in the sky. But I found that at least with the first object, that was a bonafide confirmation of a merger. And the actual what are called morphological features – so like literally the shape of the galaxy – they were all indicative of potentially merging, or already ongoing mergers. That was probably the hardest part. And so, that was definitely one of the hardest decisions I had to make. And I thought one of the things that I would come under fire for the most.
[ Back to topics ]
What other disciplines might learn from astrophysics
Watkins: Since the beginning of time, human tip look to the night sky with wonder. With both the technical and theoretical innovations of astrophysics, we now have a better – but yet far from complete – understanding of our universe. We took this opportunity to ask Arianna what she believes researchers and other disciplines – including the social sciences and humanities – might learn from our astrophysicists colleagues.
Long: One of the things that I find most fun about my work is – and I think applies to most people’s work is – when I’m looking at something, I’m looking at it for a very, very, very, very, very brief time in its entire life. And part of my job as a researcher is to try to figure out what its past was – with an understanding that there’s lots of degeneracies there, there’s lots of different ways that this object or this galaxy could come to be – and trying to tease out the things that maybe led up to this certain point in time, but always leaving room for the idea that maybe something else happened that I didn’t think of. And then … like simultaneously making the same assumptions for the future. So I can make predictions about my galaxy and my protocluster core here, assuming what we often call “closed box scenarios.” So, in my paper, I talk a lot about, “assuming a closed box scenario, these galaxies may deplete their gas reservoirs in several hundred million years.” But the universe doesn’t work like that. It’s not a closed box … or it is but the galaxies are not in a closed box, they’re going to interact with their environment. And so, what I’m getting at is: when I see something, or when I see someone, I like to think like I can make assumptions about how they got to where they are without, of course, knowing every detail, and I can make assumptions about where they’re going. But at the end of the day, like I’m never going to know the absolute truth. And that’s a good thing. So instead, let’s explore the different possibilities of what could have happened and what could happen. And that’s what I find really fun, and I think highly applicable to lots of science.
[ Back to topics ]
Women of color in astrophysics
Leigh: As if the overall number of minorities in STEM fields isn’t disheartening enough, fewer than 1% of all physics bachelor’s degrees are earned by black women, and only 22 black women hold doctorates in astronomy or related fields within physics. Especially considering that Arianna as a first generation college student, her accomplishments are all the more humbling. So to conclude our conversation Ryan and I were interested in hearing her experiences as one of the only women of color in astrophysics.
Long: There is this one paper I thought was hilarious that came out and it said, “of all the STEM degrees, physicists and astrophysicists have the highest God complexes.” And, you know, we play with the universe, like that kind of thing. And so there is this culture that’s pervasive throughout the community that is very kind of dry and serious, and highly rigorous and academic, and more focused on competition than, you know, community and connection and collaboration. And of course, you know, I have to preface everything by, “not all and not everywhere, and there are collaborations,” all that. But in general, like that is the pervasive culture. And so coming … being a woman of color coming from … even just being a person coming from a different culture, that’s not like the traditional kind of tight-lipped, tight-knit community that’s there. I mean, I laugh louder than everybody, I dress in colors, my hair is big. Like, I can’t, I can’t hide. It feels like I’m simultaneously ignored, but also I can’t hide. And so, you know, one of my go to examples is if I’m in a colloquium or seminar, and I ask a question – and I’m worried that it’s a dumb question, because I’m a grad student, I’m always worried about that – everyone always remember that it was me, I can’t hide among the masses, because I stick out like a sore thumb compared to everyone else in my community. And so that can be a little bit frustrating, this like pressure to kind of represent my culture or whatever. And it’s not … it’s, it’s never … it’s never explicitly stated. It’s kind of implied in that way. Like, I feel like I have to be careful with – or at least I used to feel like I have to be careful with – how I speak or what I dress. But I will say … how do I say this … this sudden, recent galvanizing during the COVID pandemic, and, and during Black Lives Matter this year in particular – because I’ve been involved, I’ve been passionate about these things for years, as most Black people are -the more sudden uptick has kind of put me in this place of being … I’ve been able to be a little bit more unapologetic about myself, because so many people are trying to pay more attention. They’re trying to do to do better. And that has actually felt kind of nice, because when I would bring things up before my department, or certain individuals, I would get pushback.I get kind of like swept on the rug like, “Oh, you know, we’re not really focusing on Black people right now, we’re focusing on women.” Like that kind of thing. Like in terms of recruitment, I got that a lot. And now, you know, I get emails saying, “How can we do better? Are you going to show up can can? How are you doing?” Like, “Do you have any ideas?” So it’s … there’s a sudden, slight power involved. That’s kind of nice. I don’t know how long it will last.
But you know, I think this year, we’re finally going to break 200 black women with PhDs in physics and astronomy. In all of history. This is not just like today, it’s in all of history. And I think there’s about 20- or 30-thousand people in all of history that have had PhDs in physics and astronomy. So it is a very marginalized group, for sure. And it feels … it’s this weird dichotomy of feeling on one hand, very isolated, but on the other hand, in a very, very tight-knit and supportive community. Because there’s so few of us, a lot of us know each other. It’s it’s possible for us to have Facebook groups and group chats, and actually include all Black women in physics and astronomy grad students, for example. And so a lot of us were separated from each other physically speaking on the planet. A few of my friends are fortunate enough to have each other in their departments. But for the most part, we’re usually the only ones in our departments. And that can feel awful and isolating and not great. But it also, on the flip side, it can feel really nice to know that like I can name, you know, all the grad students who are Black women in another department and reach out to them and talk to them at any point. So that’s like a plus for sure. I guess. At least that’s a silver lining I try to focus on.
[ Back to topics ]
Links to manuscript, bonus audio and other materials
Watkins: That was Arianna Long discussing her article, “Emergence of an ultra red ultra massive galaxy cluster core at z = 4,” which she published along with multiple co-authors on August 1, 2020 in the Astrophysical Journal. You’ll find a link to their article at parsingscience.org/e88 along with transcripts, bonus audio clips, and other materials we discussed during the episode.
Leigh: If you liked what you’ve been hearing on Parsing Science, then head over to wherever you might get your podcast and subscribe. And if you already do subscribe, consider leaving a review on iTunes. It’s not only a great way for others to learn about the show, it’s also a great way to help spread word of the work of the scientists on it.
[ Back to topics ]
Preview of next episode
Watkins: Next time, in episode 89 of Parsing Science, we’ll talk with Jesse Casana, from Dartmouth College about his research using multi-sensor drones to collect data about a major Native American settlement reportedly encountered in 1601 in what is now southeastern Kansas.
Jesse Casana: So in this case, what we’re trying to do is to look at the differences in the thermal properties of the soil and of the features that are on and below the ground. If you can imagine something simple like, say, a buried stone wall under the ground: that wall made of rock right is probably going to heat up and cool down at a different rate than the soil that it’s in.
Watkins: We hope that you will join us again.
[ Back to topics ]